Thank you for visiting nature.com. You are using a browser version with limited support for CSS. To obtain the best experience, we recommend you use a more up to date browser (or turn off compatibility mode in Internet Explorer). In the meantime, to ensure continued support, we are displaying the site without styles and JavaScript.
Nature Materials volume 14, pages 500–504 (2015 )Cite this article Fev
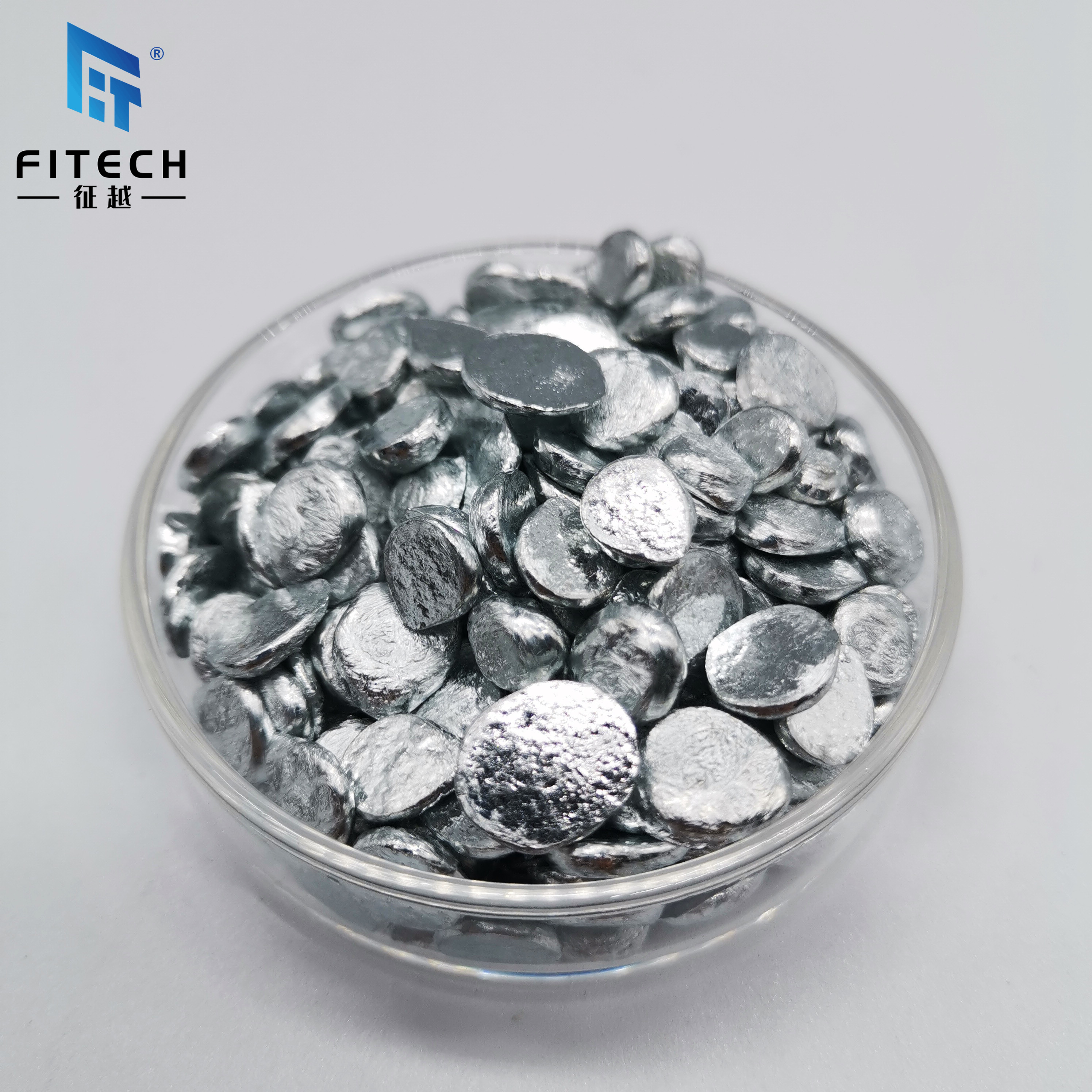
Bismuth-oxide-based materials are the building blocks for modern ferroelectrics1, multiferroics2, gas sensors3, light photocatalysts4 and fuel cells5,6. Although the cubic fluorite δ-phase of bismuth oxide (δ-Bi2O3) exhibits the highest conductivity of known solid-state oxygen ion conductors5, its instability prevents use at low temperature7,8,9,10. Here we demonstrate the possibility of stabilizing δ-Bi2O3 using highly coherent interfaces of alternating layers of Er2O3-stabilized δ-Bi2O3 and Gd2O3-doped CeO2. Remarkably, an exceptionally high chemical stability in reducing conditions and redox cycles at high temperature, usually unattainable for Bi2O3-based materials, is achieved. Even more interestingly, at low oxygen partial pressure the layered material shows anomalous high conductivity, equal or superior to pure δ-Bi2O3 in air. This suggests a strategy to design and stabilize new materials that are comprised of intrinsically unstable but high-performing component materials.
This is a preview of subscription content, access via your institution
Open Access articles citing this article.
NPG Asia Materials Open Access 24 June 2022
Nano-Micro Letters Open Access 19 June 2020
Receive 12 print issues and online access
Rent or buy this article
Prices vary by article type
Prices may be subject to local taxes which are calculated during checkout
Park, B. H. et al. Lanthanum-substituted bismuth titanate for use in non-volatile memories. Nature 401, 682–684 (1999).
Wang, J. et al. Epitaxial BiFeO3 multiferroic thin film heterostructures. Science 299, 1719–1722 (2003).
Sears, W. M. The gas-sensing properties of sintered bismuth iron molybdate catalyst. Sensors Actuators 19, 351–370 (1989).
Gurunathan, K. Photocatalytic hydrogen production using transition metal ions-doped-Bi2O3 semiconductor particles. Int. J. Hydrog. Energy 29, 933–940 (2003).
Wachsman, E. D. & Lee, K. T. Lowering the temperature of solid oxide fuel cells. Science 334, 935–939 (2011).
Azad, A. M., Larose, S. & Akbar, S. A. Bismuth oxide-based solid electrolytes for fuel cells. J. Mater. Sci. 29, 4135–4151 (1994).
Kharton, V. V., Marques, F. M. B. & Atkinson, A. Transport properties of solid oxide electrolyte ceramics: A brief review. Solid State Ion. 174, 135–149 (2004).
Shuk, P., Wiemh, H. D., Guth, U., Göpel, W. & Greenblatt, M. Oxide ion conducting solid electrolytes based on Bi2O3 . Solid State Ion. 89, 179–196 (1996).
Takahashi, T. & Iwahara, H. High oxide ion conduction in sintered oxides of the system Bi2O3-WO3 . J. Appl. Electrochem. 3, 65–72 (1973).
Sammes, N. M., Tompsett, G. A., Nafe, H. & Aldinger, F. Bismuth based oxide electrolytes-structure and ionic conductivity. J. Eur. Ceram. Soc. 19, 1801–1826 (1999).
Battle, P. D., Catlow, C. R. A., Heap, J. W. & Moroney, L. M. Structural and dynamical studies of δ-Bi2O3 oxide-ion conductors II. A structural comparison of (Bi2O3)1−x(M2O3)x for M = Y, Er and Yb. J. Solid State Chem. 67, 42–50 (1987).
Jiang, N. & Wachsman, E. D. Structural stability and conductivity of phase-stabilized cubic bismuth oxides. J. Am. Ceram. Soc. 82, 3057–3064 (1999).
Boivin, J. C. & Thomas, D. Crystal chemistry and electrical properties of bismuth-based mixed oxides. Solid State Ion. 5, 523–525 (1981).
Boyapati, S., Wachsman, E. D. & Chakoumakos, B. C. Neutron diffraction study of occupancy and positional order of oxygen ions in phase-stabilized cubic bismuth oxides. Solid State Ion. 138, 293–304 (2001).
Boyapati, S., Wachsman, E. D. & Jiang, N. Effect of oxygen sublattice ordering on interstitial transport mechanism and conductivity activation energies in phase-stabilized cubic bismuth oxide. Solid State Ion. 140, 149–160 (2001).
Switzer, J. A., Shumsky, M. G. & Bohannan, E. W. Electrodeposited ceramic single crystals. Science 284, 293–296 (1999).
Lunca Popa, P. et al. Highly oriented δ-Bi2O3 thin films stable at room temperature synthesized by reactive magnetron sputtering. J. Appl. Phys. 113, 046101 (2013).
Laurent, K., Wang, G. Y., Tusseau-Nenez, S. & Leprince-Wang, Y. Structure and conductivity studies of electrodeposited δ-Bi2O3 . Solid State Ion. 178, 1735–1739 (2008).
Wachsman, E. D., Ball, G. R., Jiang, N. & Stevenson, D. A. Structural and defect studies in solid oxide electrolytes. Solid State Ion. 52, 213–218 (1992).
Takahashi, T., Esaka, T. & Iwahara, H. Conduction in Bi2O3-based oxide ion conductor under low oxygen pressure. II. Determination of the partial electronic conductivity. J. Appl. Electrochem. 7, 303–308 (1977).
Takahashi, T., Iwahara, H. & Nagai, Y. High oxide ion conduction in sintered Bi2O3 containing SrO, CaO or La2O3 . J. Appl. Electrochem. 2, 97–104 (1972).
Park, J. Y., Yoon, H. & Wachsman, E. D. Fabrication and characterization of high-conductivity bilayered electrolytes for intermediate-temperature solid oxide fuel cells. J. Am. Ceram. Soc. 88, 2402–2408 (2005).
Maier, J. Nanoionics: Ion transport and electrochemical storage in confined systems. Nature Mater. 4, 805–815 (2005).
Steele, B. C. H. Appraisal of Ce1−yGdyO2−y/2 electrolytes for IT-SOFC operation at 500 °C. Solid State Ion. 129, 95–110 (2000).
Garcia-Barriocanal, J. et al. Colossal ionic conductivity at interfaces of epitaxial ZrO2:Y2O3/SrTiO3 heterostructures. Science 321, 676–680 (2008).
Sata, N., Eberl, K., Eberman, K. & Maier, J. Mesoscopic fast ion conduction in nanometre-scale planar heterostructures. Nature 408, 946–949 (2000).
Sanna, S. et al. Enhancement of ionic conductivity in Sm-doped ceria/yttria-stabilized zirconia heteroepitaxial structures. Small 6, 1863–1867 (2010).
Schweiger, S., Kubicek, M., Messerschmitt, F., Murer, C. & Rupp, J. L. M. A. Microdot multilayer oxide device: Let us tune the strain-ionic transport interaction. ACS Nano 8, 5032–5048 (2014).
Ni, D. W. et al. Densification of highly defective ceria by high temperatures controlled reoxidation. J. Electrochem. Soc. 161, F1–F7 (2014).
Verkerk, M. J., Van de Velde, G. M. H. & Burggraaf, A. J. Structure and ionic conductivity of Bi2O3 substituted with lanthanide oxides. J. Phys. Chem. Solids 43, 1129–1136 (1982).
The authors would like to thank C. R. Graves for valuable suggestions and discussions and for critically reading the manuscript. O. Balmes is also acknowledged for his valuable help during the experiments at beam line ID03 at the ESRF. We also appreciate the help of E. Abdellahi with the preparation of (S)TEM specimens. We gratefully acknowledge The Danish Council for Independent Research |Natural Sciences, for travel support in connection with the synchrotron experiments, through the DANSCATT grant. The A.P. Møller and Chastine Mc-Kinney Møller Foundation are gratefully acknowledged for their contribution towards the establishment of the Center for Electron Nanoscopy in the Technical University of Denmark.
Simone Sanna and Vincenzo Esposito: These authors contributed equally to this work.
Department of Energy Conversion and Storage, Technical University of Denmark, DTU Risø Campus, DK-4000 Roskilde, Denmark
Simone Sanna, Vincenzo Esposito, Jens Wenzel Andreasen, Johan Hjelm, Wei Zhang, Søren Bredmose Simonsen, Søren Linderoth & Nini Pryds
Center for Electron Nanoscopy, Technical University of Denmark Lyngby Campus, DK-2800 Kgs.Lyngby, Denmark
Department of Chemistry & iNANO, Center for Materials Crystallography, Aarhus University, DK-8000 Aarhus C, Denmark
You can also search for this author in PubMed Google Scholar
You can also search for this author in PubMed Google Scholar
You can also search for this author in PubMed Google Scholar
You can also search for this author in PubMed Google Scholar
You can also search for this author in PubMed Google Scholar
You can also search for this author in PubMed Google Scholar
You can also search for this author in PubMed Google Scholar
You can also search for this author in PubMed Google Scholar
You can also search for this author in PubMed Google Scholar
You can also search for this author in PubMed Google Scholar
S.S., V.E., S.L. and N.P. designed this project. V.E. elaborated the concept and S.S. developed the heterostructure layered architectures. S.S. deposited the thin films by PLD. S.S. and J.W.A. performed structural characterization by XRD and synchrotron light experiments. S.S. and M.C. performed structural characterization by high-temperature XRD. W.Z., T.K. and S.B.S. performed STEM and HRTEM characterizations. S.S., V.E. and J.H. performed the electrical characterization. S.S., V.E. and N.P. analysed the data and wrote the manuscript.
The authors declare no competing financial interests.
Sanna, S., Esposito, V., Andreasen, J. et al. Enhancement of the chemical stability in confined δ-Bi2O3. Nature Mater 14, 500–504 (2015). https://doi.org/10.1038/nmat4266
DOI: https://doi.org/10.1038/nmat4266
Anyone you share the following link with will be able to read this content:
Sorry, a shareable link is not currently available for this article.
Provided by the Springer Nature SharedIt content-sharing initiative
Journal of Materials Science: Materials in Electronics (2023)
Journal of Materials Science: Materials in Electronics (2022)
Nature Materials (Nat. Mater.) ISSN 1476-4660 (online) ISSN 1476-1122 (print)
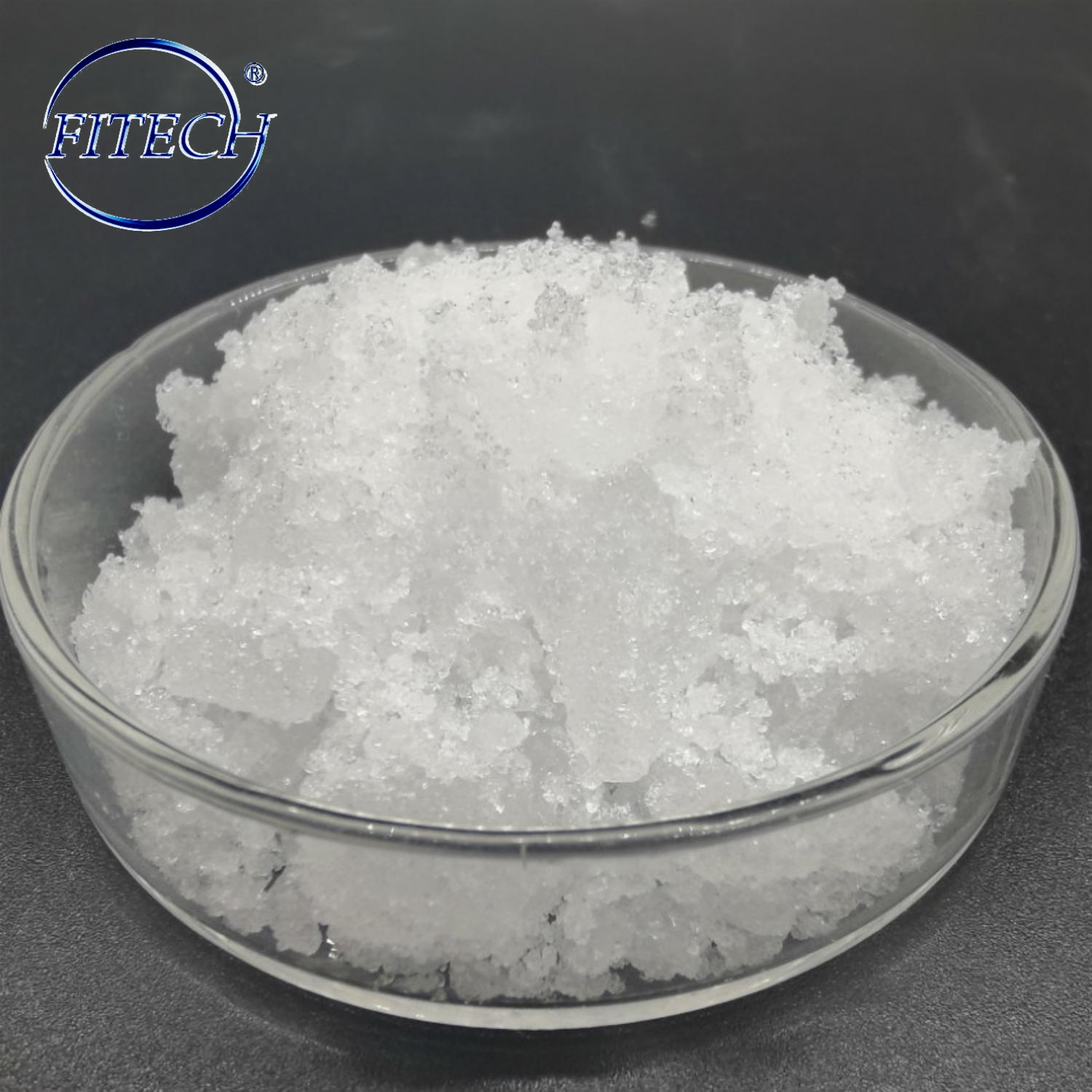
Ferrovanadium Sign up for the Nature Briefing newsletter — what matters in science, free to your inbox daily.